Scientific Background
​
Various microalgae species have the ability of producing gaseous hydrogen during the process of photosynthesis, using a special enzyme called hydrogenase which upon reduction catalyzes the following reaction:
​
2 protons + 2 electrons -> Hydrogen
​
However, the amount of hydrogen produced naturally is insignificant.
There are 2 major bottlenecks that limit the efficiency of the natural hydrogen production process:
1) Electron diversion: over 85% of the electrons derived from water splitting at photosystem II are channeled to competing processes, mainly carbon fixation.
2) Oxygen sensitivity: when exposed to oxygen, the hydrogenase enzyme undergoes inactivation.
Thus, in order for hydrogen photo-production in algae to become a viable source for clean energy we must engineer new algal strains in which a substantial fraction of photosynthesis-derived electrons will be shuttled towards active hydrogenase enzymes.
​
Our research
​
To bioengineer more efficient synthetic enzymes, we are exploring energy (electron transfer) junctions. For example, the fact that all outgoing energy-transfer from the photosystem I is solely mediated by ferredoxin is being exploited. We hypothesized that a physical fusion of the energy carrier component (Ferredoxin) with the hydrogen producing catalyst (Hydrogenase) could be the key to bypass electron divergence towards carbon fixation. The physical proximity of the two proteins during photosynthesis results in immediate energy transfer from ferredoxin to hydrogenase, which could then be used for hydrogen production. In our Lab, additional junctions are being studied and novel bypasses are being developed.
We are genetically engineering different algal strains to stably and efficiently produce hydrogen under an array of experimental settings. We are using state of the art equipment to transform, grow and monitor the parameters of interest. However, the entire field of algae biotechnology is hindered by the difficulty of achieving algal strains with high levels of heterologous expression. To this end we are applying cutting edge computational methods to model the process of gene expression in algae. We are specifically interested in developing sequence optimization algorithms and studying the evolution of the chloroplast genome.
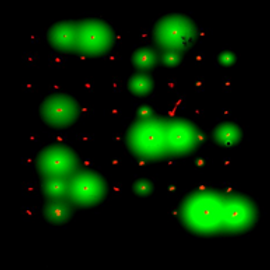
Rhodobacter high throughput screening system
Algal clones overlaid with hydrogen sensing R. capsulatus. These bacteria produce GFP in the presence of hydrogen, thus the green halation represents accumulated hydrogen whereas the red halation is the colonies’ chlorophyll fluorescence. In this experiment the production of hydrogen signifies a successful insertion of an engineered hydrogenase enzyme into the native alga’s genome.
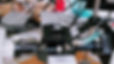
Membrane inlet mass spectrometer coupled to a pulse amplitude modulation system
​
Algae in liquid solution is inserted into the glass cuvette and simultaneously monitored in real-time for a variety of photosynthetic parameters and other metabolic activities. These include the production and consumption of an array of gasses (e.g. hydrogen, oxygen, CO etc.) and electron transfer rates.
​
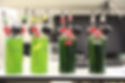
2
Photo-bioreactors
In this modulated system algal strains are grown under controlled conditions and continuously monitored in real-time for hydrogen production, colony density and other essential parameters. We typically conduct long-term experiments in these bioreactors in which the accumulated biologically produced hydrogen is stored over time.

Unsupervised machine learning
In out attempts to increase the expression levels of our synthetic enzymes in living algal cells, we use different learning algorithms to decipher the information governing the regulation of gene expression in microalgae. In this clustering analysis we were looking for patterns which could explain the presence or absence of canonical prokaryotic translation mechanisms in the chloroplast genome.